Purpose
Interventional radiotherapy (IRT, brachytherapy) is a highly effective treatment method for non-melanoma skin cancer (NMSC). It is characterized by excellent functional and cosmetic outcomes, and since it is performed on an outpatient basis, treatment costs are generally low [1]. For such reasons, there is a growing interest among radiation oncologists and dermatologists in this treatment modality [2]. In 2015, the American Brachytherapy Society (ABS) working group released a report recommending not to exceed 5 mm in depth prescription for NMSC with contact IRT (cIRT) to prevent unacceptable skin-surface dose and reduce toxicity [3]. Similar indications about the depth prescription were published by the GEC-ESTRO recommendations for the skin in 2018 [4].
In accordance with these recommendations, in cases with tumor thickness > 5 mm, interstitial IRT or sometimes, a combination of interstitial and cIRT approach can be performed [5]. However, when we consider the evidence of surveys in clinical practice, there is a more complex scenario, where the actual depth of prescription greatly varies from center to center and across different countries [6]. A survey performed among members of the ABS revealed that the median maximum depth of prescription was actually 5 mm, but ranging from 1 to 8 mm [7].
In 2022, the H&N and Skin (HNS) GEC-ESTRO working group performed a systematic review of literature confirming these variations among institutions, and suggesting that it is acceptable to prescribe cIRT dose at a depth above 5 mm beyond the skin surface [8].
In our clinical experience, contact IRT plays a major role in the treatment of NMSC located in critical areas, in which functional and cosmetic results are crucial, such as the face, where surgical approach may have potentially disfiguring consequences [9]. cIRT has been demonstrated as an effective therapeutic option, especially within a pandemic clinical scenario, due to the possibility to treat patients with hypofractionated schedules that reduce the total number of hospital admissions necessary to complete the treatment [10].
When it comes to deal with toxicity in NMSC after cIRT, it is important to carefully consider several factors, including patient’s pre-existing comorbidities of (vascular problems, diabetes, etc.) or anatomical site treated, and issues related to IRT treatment, such as total dose delivered, fractionation schedule, and dosimetric parameters (dose to the skin) [11]. There is no definitive agreement among researchers about the optimal parameter to consider; however, in a recent survey among ABS members, the maximum acceptable dose to the skin was 150% [7].
Based on these premises, it is extremely important to underline the emerging role of pre-treatment imaging, which is rapidly growing in the clinical practice. Furthermore, the use of ultrasound allows for accurate determination of the precise measures of NMSC lesion, reaching local control rates of up to 99.3% [12].
The aim of the current paper was to describe a multilayer arrangement of catheters to treat NMSC lesions thicker than 5 mm while sparing the excess of dose to the skin surface.
Material and methods
A solid water phantom was used for the purpose of this study, and a CT scan was obtained with a slice thickness of 0.625 mm on a Discovery CT590RT CT scanner (GE Medical System). OncentraBrachy treatment planning system (TPS v.4.6.2 Elekta, Sweden) was applied for organs at risk (OARs) and clinical target volume (CTV) contouring and planning. Normally, the term ‘therapeutic window’ (TW) is referred to as the separation between tumor control and adverse event in clinical setting. In skin IRT, tumor control is strictly related to the coverage with 100% isoline (dose prescription) and the maximum isoline allowed to prevent toxicity, which in several series is reported as high as 150% isoline [7, 9]. For such reason in this report, we referred to the TW as the distance between the 100% and the 150% isoline.
In order to determine how the TW changes with the catheter distance from the skin, 21 treatment plans were performed using a single-source, with distances from the center of the catheter to the skin varying from 1.9 to 53.1 mm. This analysis was done as a theoretical approach to the optimization of skin treatment plans. However, distances of more than 2 or 3 cm are considered not feasible in the clinical practice, as the implant would be far from the patient and more prone to positional errors. Therefore, a multilayer catheter arrangement was introduced to investigate how to best optimize TW in the clinical practice, even for lesions with different thickness. A second CT scan was acquired using two Freiburg flap applicators (Elekta, Sweden) placed one on top of the other on the outer surface of the phantom. Each Freiburg flap has a constant distance of 5 mm from the center of treatment catheter to the surface, with treatment channels 10 mm apart from each other. External skin of the phantom was contoured, and ad hoc CTV was created (35 × 35 mm, and 5 and 8 mm thick) as shown in Figure 1. Five catheters were activated in two different arrangements. Standard configuration was prepared with 5 catheters in the lower flap only (Figure 1A) according to the standard use of Freiburg flap. Multilayer configuration was done with 5 catheters distributed within two flaps, as shown in Figure 1B. For both configurations, 325 active dwell positions were applied. Treatment plans were graphically optimized by an experienced planner, so that 150% isoline would be tangential to the skin and V150 (CTV) would be equal to 1.5% for both the configurations. In this study, dose calculations for iridium-192 high-dose-rate (HDR) source were performed with the TG-43 formalism [13].
Fig. 1
Standard configuration of catheters (A) and multilayer configuration (B) calculated with the TG-43 formalism. 150%, 100%, 95%, and 80% isolines for the two configurations are compared. Treatment plans are optimized with 150% tangential to the skin, with a value of V150 (CTV) set to 1.5% for both the configurations
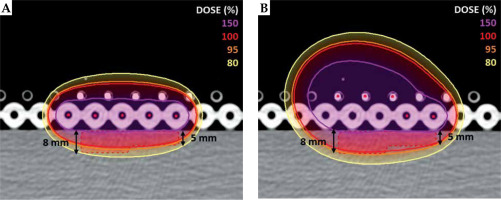
Results
For single-source configuration, the TW was found to linearly increase with the catheter to the skin distance (Figure 2), with a range between 0.6 and 11.7 mm for catheter-to-skin distances between 1.9 and 53.1 mm. Figure 1 shows comparison between the standard and multilayer configurations. The standard configuration with 5 activated catheters in the lower flap resulted in CTV coverage of V95 (CTV) equal to 81.84%, whilst the multilayer configuration resulted in a V95 (CTV) equal to 95.68%, with a V150 (CTV) set to 1.5% for both the configurations. The treatment time was 3.12 minutes for the standard configuration and 4.79 minutes for the multilayer configuration.
Fig. 2
Therapeutic window (TW) as a function of catheter-to-skin distance (mm) for a single active dwell position. TW increases in the depth, with values ranging from 0.6 to 11.7 mm for catheter-to-skin distances between 1.9 and 53.1 mm. Error bars show a 0.2 mm error for the distance measurement on TPS
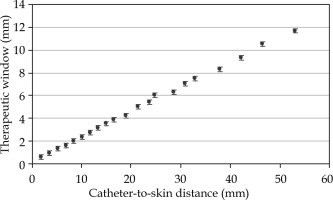
Discussion
In scientific literature, several authors have extensively analyzed main differences between the static and dynamic intensity modulated IRT [14-16]. In Table 1, it is possible to compare an example of static intensity modulated IRT for uveal melanoma using plaques with iodine-125 seeds [17, 18] with dynamic IRT, either HDR or pulsed-dose-rate (PDR), by focusing on three main factors, such as space, time, and intensity, which are commonly used in the clinical practice to modulate dose distribution. Regarding intensity, a modulation in this case could be achieved by using different catheter-to-skin distances in case of dynamic intensity modulated IRT.
Table 1
Differences between static and dynamic intensity modulated interventional radiotherapy (IRT)
These factors, combined together, play a pivotal role in modulating the TW, which means adequately covering the entire depth of the lesion, with the prescription dose simultaneously avoiding the excess of the dose to the surface of the skin above.
As it is possible to see from Table 1, the only factor that in dynamic intensity modulated IRT is probably still underused in the clinical practice, is the catheter-to-skin distance according to the thickness of treated lesion. In particular, for lesions thicker than 5 mm, the use of this additional parameter is of paramount relevance, because it allows to obtain a more favorable dosimetric distribution with a consequent increase in terms of TW. In fact, results of a survey performed among ABS members showed that most radiation oncologists use a catheter-to-skin distance ranging from 5 to 10 mm [7]. Slightly different results were obtained from a survey performed in Canada, where a catheter-to-skin distance was reported to vary between 2.5 and 15 mm [6].
It is important to mention that even though the traditional name of interventional radiotherapy, i.e., brachytherapy, comes from ancient Greek word ‘brachy’ means ‘short’, thus underlining that main feature of brachytherapy is to place sources as near as possible to target volume, in our analysis, we came to a conclusion that adding further layers of sources far from the target volume using a multilayer spatial distribution, may contribute to obtain a better local dose distribution within the target volume itself. This is due to the possibility of modulating the dose when using multiple layers of catheters, taking advantage of a larger TW for bigger distances from the source. This is particularly important when comparing IRT with external beam radiotherapy (EBRT), where the modulation of dose is achievable, for instance, by multi-leaf collimators and machine movements that are not available in IRT. The advantages of IRT over EBRT have already been investigated elsewhere [19], but the more efficient modulation described in our study may further support the use of IRT, especially in treatment of lesions with varying thickness or when the lesions are situated in concave or convex areas.
Our results showed that catheter placement at varying depths from the treatment surface might better modulate dose distribution and obtain a greater CTV coverage without increasing hot spots. A greater coverage involves a small increase in treatment time and not clinically significant increase dose to deep healthy tissue. It is however necessary, on the basis of image-guided procedures, to verify the dose to organs at risk and optimize the distribution according to constraints.
The results obtained in our analysis may be used in several clinical applications, including Freiburg flaps, and individualized moulds either manually manufactured or 3D-printed [20]. In particular, there is a possibility of designing multi-depth catheter placements based on lesion thickness in 3D-printed applicators. Future improvements may include a combination of 3D printing and artificial intelligence, which is expected to reduce the time for mould preparation, allowing to treat areas with vast anatomical difficulties [21, 22].
Since IRT for skin cancer is not routinely performed in all centers, it is important to generate more solid evidence both by promoting prospective trials and collecting real-life data within the frame of shared ontology, which allows to better compare the results obtained [23, 24].